LepVax as a Promising Specific Vaccine for Leprosy: A Narrative Review
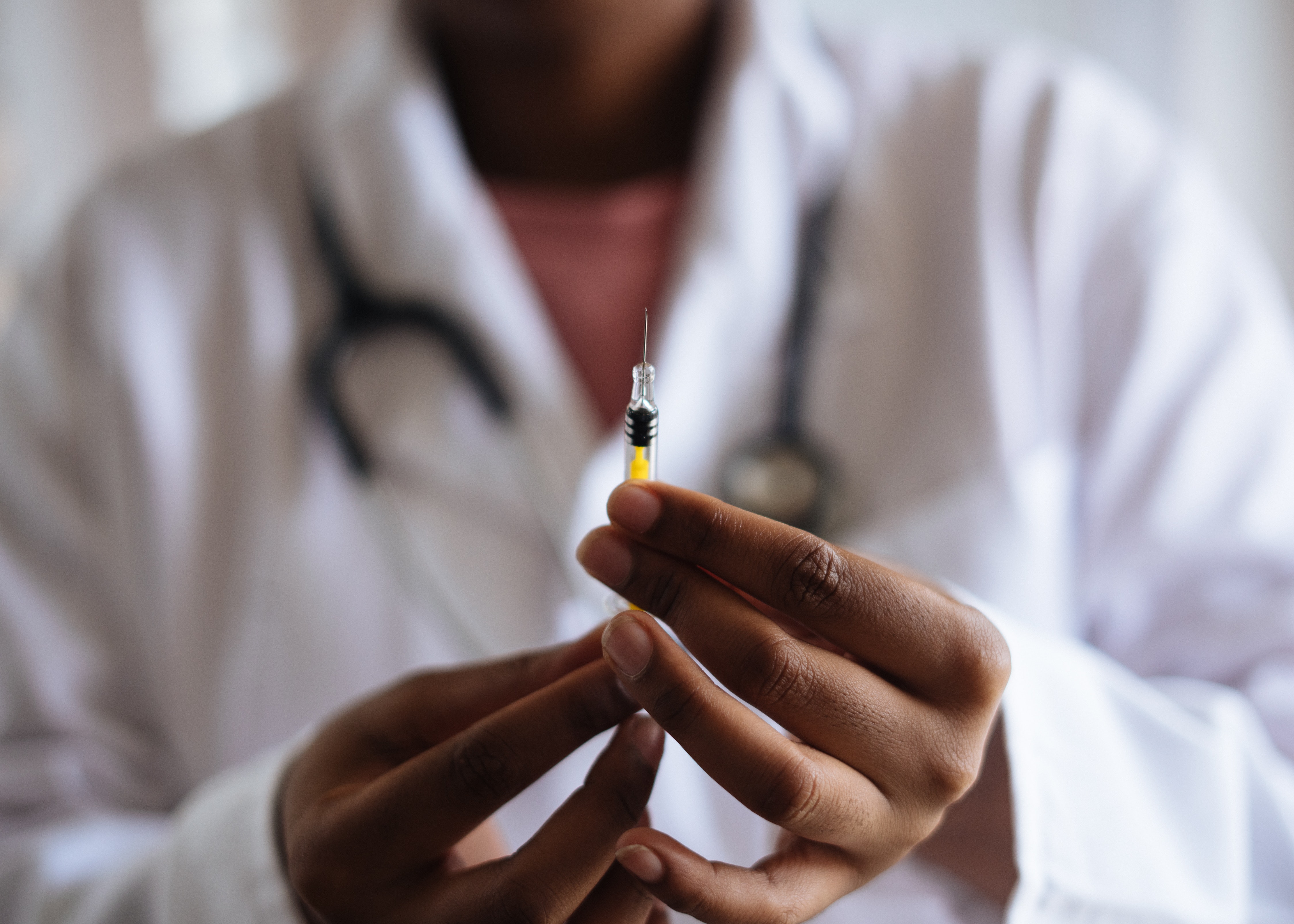
Abstract
Leprosy is a disease with a high rate of transmission around the world. Leprosy is hard to eradicate because treatment is difficult and there is no vaccine to prevent it. The response of the immune system plays a role in determining the form of the clinical manifestation of leprosy. LepVax is a vaccine made from the Mycobacterium leprae antigens ML2531, ML2380, ML2055 and ML2028 formulated with the Toll-like receptor 4 agonist Glucopyranosyl Lipid Adjuvant in Stable Emulsion. LepVax can prevent infection with M. leprae through stimulation of the immune system and resulting regulation of pathogenic bacteria. LepVax can prevent nerve cell damage which is a target of the M. leprae bacterium. Further research is needed on the safety of LepVax as a vaccine to eradicate leprosy in the future.
Introduction
Leprosy, also known as Morbus Hansen, is a contagious infectious disease with a high incidence of transmission worldwide. Based on reports from the World Health Organization, in 2017 there were 210,671 reported cases of leprosy worldwide with 192,713 cases currently being treated. The regions with the highest incidence in the world are Southeast Asia (153,487 cases), the Americas (29,101 cases) and Africa (20,416 cases) [1]. Leprosy also caused Years Lived with Disability (YLDs) to reach 31,500 in 2017 [2]. Leprosy is caused by the bacillus Mycobacterium leprae which is a type of aerobic bacteria that forms bacilli and has acid-resistant properties. The pathogen M. leprae can be transmitted through nasal droplets which can last for nine days outside the human body or through direct contact with leprosy patients. Pathogen transmission is also possible via blood transfusion, vertical transmission, breast milk and vector transmission but these cases are less common. M. leprae can also be transmitted through armadillos, mostly in southern USA. A complication of leprosy is erythema nodosum leprosum (ENL) which is caused by high levels of proinflammatory cytokines producing inflammation throughout the patient's body [3]. M. leprae bacteria infect the skin, eyes, nasal mucosa and peripheral nervous system. M. leprae bacteria have a predilection towards Schwann cells and macrophages in the skin. The initial manifestations of leprosy depend on the immune response. Hypopigmented white spots and neuropathy causing sensory loss in the area of infection lead to skin lesions that are not detected until they manifest causing disability [4].
Leprosy therapy currently uses various types of drug therapy/Multi-Drug Therapy (MDT) which was introduced by WHO in 1982. Drugs given to leprosy patients include dapsone, clofazimine and rifampicin, which need to be given for 6-12 months. Some of the side effects of these medicines include hepatotoxicity, nausea, vomiting and fever. Also, there are some drugs such as clofazimine which are contraindicated in patients with kidney failure and can cause skin discolouration [4]. MDT treatment has contributed to reducing the prevalence of leprosy from 0.32 per 10,000 population in 2014 to 0.29 per 10,000 in 2016 [5]. However, MDT administration has not been able to eliminate leprosy. This is because there are patients who drop out of treatment in areas where leprosy is endemic. Several factors such as side effects from MDT, inaccessibility of remote health facilities and stigma that have an impact on the psychological, social and economic factors of patients can contribute to the neglect of treatment of leprosy [6]. Besides, the efficacy of MDT treatment is limited to cases of low bacterial load (Bacillary Index (BI) ≤4 +) with high relapse rates and high costs due to long treatment periods making it less effective at eliminating leprosy.[7]
The administration of the Bacille Calmette-Guerin (BCG) vaccine is a preventative action that can be taken to reduce the incidence of leprosy. The BCG vaccine, besides preventing leprosy, can also prevent tuberculosis (TB) infection due to the bacterium Mycobacterium tuberculosis. Several studies have shown that some BCG vaccines can reduce TB incidence by 83%, but BCG vaccines are not able to reduce the incidence of leprosy effectively [8]. Therefore, there is a need for a vaccine to eradicate leprosy in the community. One of the developments of a vaccine for leprosy is LepVax, a leprosy vaccine formulated from M. leprae bacterial antigens in combination with Toll-like receptor 4 (TLR4) agonist which has the potential to prevent leprosy [9]. The aim of this review will be to discuss the potential of LepVax as a leprosy vaccine and its mechanism in the body for the prevention of leprosy infection.
Immune Response and Pathogenesis of Leprosy
M. leprae bacteria spread through the lymph to Schwann cells and bind to the basal lamina. Because Schwann cells have inadequate lysosome enzymes, pathogens survive in Schwann cells and continue to multiply. In the long term, these pathogens spread to the dermis layer around the superficial plexus of blood vessels and cause lesions due to the accumulation of pathogens in the skin. The pattern of leprosy and the development of clinical manifestations of the disease will vary according to both host immune system factors and the pathogenicity of M. leprae. With good immunity and an avirulent pathogen, the body can become immune, resulting in no clinical manifestations of infection. Clinical manifestations in patients can depend on the reaction between Th1 cells and Th2 cells of the immune system. In the immune response of Th1 cells, decreased bacterial activity is achieved through the production of TNF-α and INF-γ and activation of Th17 cells which activate macrophages and promote microbicidal activity, thereby destroying bacteria. The dominance of the Th1 immune system can localise pathogenic bacteria leading to healing or the formation of localised disease in the form of paucibacillary (PB) skin lesions, which in the extreme form are termed tuberculoid leprosy/TT. In the Th2 immune response, release of cytokines IL-4, IL-10, and TGF-β and activation of Treg cells inactivate the microbicidal response of macrophages, thereby facilitating the survival of bacilli and allowing bacteria to replicate to produce a multibacillary (MB) skin lesion which in its extreme form is termed lepromatous leprosy/LL [10]. The majority of leprosy patients show pathogenesis somewhere inbetween and were classified by Ridley Joplin criteria as borderline tuberculoid (BT) or borderline lepromatous (BL) which involves and immunologically unstable host response to the M. leprae antigen [11]. The finding that the activation of memory CD4 cells in recognition of pathogens stimulates Th1 cell activity has implications for protection against mycobacteria [12].
Development of LepVax as a Leprosy Vaccine
LepVax, a leprosy vaccine derived from the M. leprae antigens ML2531, ML 2380, ML2055 and ML2028 formulated with Toll-like receptor 4 (TLR4) agonists as adjuvant is the latest breakthrough in research on leprosy vaccines [8]. The use of bacterial antigens with a TLR agonist can initiate innate immune responses in recognising pathogens so that the correct immune response is formed to prevent infection with CD4 + T cell maturation, proliferation and differentiation [13]. Modulation of the immune response through activation of T cells using M. leprae antigens can help increase the body's protective effect against infection [14]. Modulation of CD4 + T cell to release IFN-γ can also promote multifunctional Th1 cells with the production of proinflammatory cytokines such as IL-1β, IL-2, IL-4, IL-5, IL-6, IL-7, IL-17 and TNF-α, which provide a protective response to leprosy infection [15].
The antigenic component of LepVax is LEP-F1, a combination of various recombinant fusion proteins from M. leprae including ML2531, ML2380, ML2055, and ML2028 antigens [16]. The bacterium Escherichia coli strain BL21is genetically engineered to produce these recombinant antigens, and is advantageous for antigen production because it is easy to culture and replicates quickly [17]. This approach makes it easier to develop the correct the amino acid chain structure, thus facilitating the generation of the specific immunoglobulin response. [18].
ML2531, ML2380, ML2055 and ML2028 antigens are widely found in paucibacillary type leprosy patients who can produce IFN-γ [19]. Research conducted by Bezerra-Santos et al (2018) found that production of IFN-γ together with IL-2 and TNF-γ from CD4 cells had a role in regenerating the effector memory of healthy household contacts (HHC) to induce more effective multifunction T cell response to M. leprae infection and to prevent the development of leprosy [20]. IFN-γ cytokines also activate CD8 cells to initiate the production of IL-2 cytokines to promote the memory function of CD8 cells and produce anti-inflammatory cytokines IL-10 to offset the inflammatory reaction [14,15]. The use of a combination of antigens can increase the availability of epitopes in the process of proliferation and differentiation of T cells, producing higher IFN-γ cytokines. Availability of IFN-γ in serum shows the high-antigen specific protective response to M. leprae infection [21].
The current postgenomic approach shows the role of ML2531, ML2380, ML2055 and ML2028 antigens in bacterial cell growth which can be determined as clinical diagnostic approaches. ML2531 antigen acts as a virulence factor mediating pathogenic reactions with T cells while ML2380 is an indicator protein antigen that is often used in serological detection. ML2055 is a protein that forms the surface layer of bacterial cells whereas the ML2028 antigen is a protein catalyst for a protective effect on the mycobacterium membrane [22]. Antigens from M. leprae enhance the immune response of the host body by stimulating the release of cytokines and chemokines from T cells. The antimicrobial response begins with the recognition of M. leprae antigens which initiate the recruitment of proinflammatory cytokines based on the recognition of M. leprae antigens with the formation of macrophages from monocytes by IFN-γ. Then, the process of phagocytosis is initiated based on the recognition of the M. leprae antigen which initiates an antimicrobial response as an innate immune response against the target.[19,23].
Lepvax Activity in Prevention of Leprosy Infection
Vaccine immunogenicity studies show the vaccine's ability to induce immunity is based on the vaccine's ability to interact with CD4 T helper cells. This interaction can release Th1 cell-type cytokines that play an important role in controlling mycobacterial infections [8]. Reaction with Th1 cells can limit the development of pathogens by localizing infection through the production of the cytokine IL-8 which in turn can induce TNF-α release from Schwann cells which are the site of pathogen invasion [19]. The presence of an immunological response from the presence of cytokines in Schwann cells means pathogenic infections can be localized and not spread to other target locations. Induction of cytokines can improve nerve function when infected by M. leprae and prevent damage to Schwann cells due to infection [24].
Vaccine reaction with Th1 cells can be initiated by using TLR4 synthesis formulation in the form of Glucopyranosyl Lipid Adjuvant in Stable Emulsion (GLA-SE). Research conducted by Cauwelaert et al (2015) shows that GLA-SE can induce lymphoid activity to produce IFN-α which has the same production pathway as IFN-γ in lymph nodes [25]. A study conducted by Duthie et al (2014) shows that administration of GLA-SE can reduce the number of M. leprae bacteria in lymph nodes compared to controls [26]. GLA-SE interactions in the body induce multifunctional CD4 T cells in response to specific antigens with humoral immune responses. The addition of GLA-SE in vaccines provides a long-term protective effect against pathogenic infections where infections caused by pathogens cause oxidative stress on cell DNA [27,28]. The finding of the protective effect of GLA-SE can reduce the risk of cell DNA damage due to infection shows the effectiveness of GLA-SE to prevent the spread of infection to other organs and increase the prolongation of survival [28].
Studies conducted by Duthie et al (2018) show the effectiveness of Lepvax to prevent M. leprae infection by maintaining motor nerve function better than the BCG vaccine. Studies conducted with armadillos (Dasypus novemcitus) show the effect of LepVax can inhibit pathogenic infections up to 24 months post-exposure when compared with the BCG vaccine shown by deficit of compound muscle action potential (CMAP) and nerve conduction velocity (NCV) on armadillos with LepVax lower when compared with the BCG vaccine after exposure [29]. Nerve damage to Schwann cells due to M. leprae infection causes Schwann cells to change into progenitor / stem-like cell forms which cause pathogens to spread to other organs or skeletal muscles [30]. Stimulation of the immune system by administering vaccines by regulating T regulatory cells can produce immunity against pathogenic reactions that can result in more severe damage due to antimicrobial reactions in the body. [31].
The activity of the pathogenic M. leprae that proliferates in Schwann cells is supported by the peripheral environment of the body which is located in environments of low oxygen tension so that it can colonize the area of infection and cause nerve ischemia resulting in axonal damage [32]. The effect is the onset of neuropathy in the infected area as indicated by a decrease in CMAP and damage to sensory tissue. LepVax vaccine can maintain CMAP in motor nerves and prevent nerve damage due to M. leprae infection through preventing damage to Schwann cells which can disrupt the electrical conduction of nerves and restore the form of Schwann cells to normal conditions [29].
Conclusion
Leprosy caused by Mycobacterium leprae infection has become one of the infectious diseases which is one of the serious problems in the world today due to the development of the more widespread disease. At present, one of the preventions of leprosy infection through immunization with BCG vaccine is often used to vaccinate tuberculosis. However, its use as a leprosy vaccine still has low effectiveness and efficacy, so there is a need for a leprosy vaccine that has high efficacy and effectiveness. LepVax consists of M. leprae antigen with Toll-like receptor-4 (TLR4) agonist formulation to stimulate the immune system. LepVax works by stimulating the immune response to the M. leprae antigen to form an immune memory from the immune system to prevent severe leprosy infection and further nerve damage. At present, there is still a need for further clinical trials in humans and the dosages needed for LepVax to prevent leprosy infections and eradicate leprosy in the future.
References
1. World Health Organization. Global leprosy update, 2017: reducing the disease burden due to leprosy. Vol. 93, Weekly Epidemiological Record. 2018.
2. James SL, Abate D, Abate KH, Abay SM, Abbafati C, Abbasi N, et al. Global, regional, and national incidence, prevalence, and years lived with disability for 354 Diseases and Injuries for 195 countries and territories, 1990-2017: A systematic analysis for the Global Burden of Disease Study 2017. Lancet. 2018;392(10159):1789–858.
3. Lastória JC, Abreu MA. Leprosy: review of epidemiological, clinical, and etiopathogenic aspects - Part1. An Bras Dermatol. 2014;89(2):205–18.
4. Eichelmann K, González González SE, Salas-Alanis JC, Ocampo-Candiani J. Leprosy. An update: Definition, pathogenesis, classification, diagnosis, and treatment. Actas Dermosifiliogr [Internet]. 2013;104(7):554–63.
5. Al Awaidy ST. Progress towards a leprosy-free country: the experience of Oman. PLoS Negl Trop Dis. 2017;11(11):1–14.
6. Girão RJS, Soares NLR, Pinheiro JV, Oliveira GDP, De Carvalho SMF, De Abreu LC, et al. Leprosy treatment dropout: A sistematic review. Int Arch Med. 2013;6(1):1–9.
7. Malathy M, Thappa DM. Fixed-duration therapy in leprosy: Limitations and opportunities. Indian J Dermatol. 2013;58(2):93–100.
8. Coppola M, van den Eeden SJF, Robbins N, Wilson L, Franken KLMC, Adams LB, et al. Vaccines for leprosy and tuberculosis: Opportunities for shared research, development, and application. Front Immunol. 2018;9(FEB):1–12.
9. Duthie MS, Frevol A, Day T, Coler RN, Vergara J, Rolf T, et al. A phase 1 antigen dose escalation trial to evaluate safety, tolerability and immunogenicity of the leprosy vaccine candidate LepVax (LEP-F1 + GLA–SE) in healthy adults. Vaccine [Internet]. 2020;38(7):1700–7.
10. de Sousa JR, Sotto MN, Quaresma JAS. Leprosy as a complex infection: Breakdown of the Th1 and Th2 immune paradigm in the immunopathogenesis of the disease. Front Immunol. 2017;8(NOV):18–21.
11. Sadhu S, Mitra DK. Emerging concepts of adaptive immunity in leprosy. Front Immunol. 2018;9(APR):1–7.
12. de Carvalho FM, Rodrigues LS, Duppre NC, Alvim IMP, Ribeiro-Alves M, Pinheiro RO, et al. Interruption of persistent exposure to leprosy combined or not with recent BCG vaccination enhances the response to Mycobacterium leprae specific antigens. PLoS Negl Trop Dis. 2017;11(5):1–16.
13. Behzad H, Huckriede ALW, Haynes L, Gentleman B, Coyle K, Wilschut JC, et al. GLA-SE, a synthetic toll-like receptor 4 agonist, enhances T-cell responses to influenza vaccine in older adults. J Infect Dis. 2012;205(3):466–73.
14. Bezerra-Santos M, do Vale-Simon M, Barreto AS, Cazzaniga RA, de Oliveira DT, Barrios MR, et al. Mycobacterium leprae Recombinant Antigen Induces High Expression of Multifunction T Lymphocytes and Is Promising as a Specific Vaccine for Leprosy. Front Immunol. 2018;9(December):2920.
15. Oliveira RM, Hungria EM, De Araújo Freitas A, De Sousa ALOM, Costa MB, Reed SG, et al. Synergistic antigen combinations for the development of interferon gamma release assays for paucibacillary leprosy. Eur J Clin Microbiol Infect Dis. 2014;33(8):1415–24.
16. Duthie MS, Casper C, Reed SG. Second coming: the re-emergence and modernization of immunotherapy by vaccines as a component of leprosy control. Future Microbiol. 2018;13(13):1449–51.
17. Rosano GL, Ceccarelli EA. Recombinant protein expression in Escherichia coli: Advances and challenges. Front Microbiol. 2014;5(APR):1–17.
18. Barbosa M dos S, de Sousa IBA, Simionatto S, Borsuk S, Marchioro SB. Recombinant polypeptide of Mycobacterium leprae as a potential tool for serological detection of leprosy. AMB Express [Internet]. 2019;9(1).
19. Chen X, You Y-G, Yuan Y-H, Yuan LC, Wen Y. Host immune responses induced by specific Mycobacterium leprae antigens in an overnight whole-blood assay correlate with the diagnosis of paucibacillary leprosy patients in China. PLoS Negl Trop Dis [Internet]. 2019;13(4):e0007318.
20. Sampaio LH, Stefani MMA, Oliveira RM, Sousa ALM, Ireton GC, Reed SG, et al. Immunologically reactive M. leprae antigens with relevance to diagnosis and vaccine development. BMC Infect Dis. 2011;11.
21. Duthie MS, Balagon MF. Combination chemoprophylaxis and immunoprophylaxis in reducing the incidence of leprosy. Risk Manag Healthc Policy. 2016;9:43–53.
22. British Leprosy Relief Association. Postgenomic Mycobacterium leprae antigens for cellular and serological diagnosis of M. leprae exposure, infection and leprosy disease. The Free Library. 2011.
23. Pinheiro RO, Schmitz V, de Andrade Silva BJ, Dias AA, de Souza BJ, de Mattos Barbosa MG, et al. Innate immune responses in leprosy. Front Immunol. 2018;9(MAR):1–15.
24. Hagge DA, Scollard DM, Ray NA, Marks VT, Deming AT, Spencer JS, et al. IL-10 and NOS2 Modulate Antigen-Specific Reactivity and Nerve Infiltration by T Cells in Experimental Leprosy. PLoS Negl Trop Dis. 2014;8(9):1–13.
25. Cauwelaert ND, Desbien AL, Hudson TE, Pine SO, Reed SG, Coler RN, et al. The TLR4 agonist vaccine adjuvant, GLA-SE, requires canonical and atypical mechanisms of action for TH1 Induction. PLoS One. 2016;11(1):1–14.
26. Duthie MS, Coler RN, Laurance JD, Sampaio LH, Oliveira RM, Sousa ALM, et al. Protection against Mycobacterium leprae Infection by the ID83/GLA-SE and ID93/GLA-SE Vaccines Developed for Tuberculosis. Infect Immun. 2014;82(9):3979–85.
27. Kwon KW, Lee A, Larsen SE, Baldwin SL, Coler RN, Reed SG, et al. Long-term protective efficacy with a BCG-prime ID93/GLA-SE boost regimen against the hyper-virulent Mycobacterium tuberculosis strain K in a mouse model. Sci Rep. 2019;9(1):1–14.
28. Baldwin SL, Reese VA, Huang PWD, Beebe EA, Podell BK, Reed SG, et al. Protection and Long-Lived Immunity Induced by the ID93/GLA-SE Vaccine Candidate against a Clinical Mycobacterium tuberculosis Isolate. Clin Vaccine Immunol. 2016;23(2):137–47.
29. Duthie MS, Pena MT, Ebenezer GJ, Gillis TP, Sharma R, Cunningham K, et al. LepVax, a defined subunit vaccine that provides effective pre-exposure and post-exposure prophylaxis of M. leprae infection. npj Vaccines [Internet]. 2018;3(1).
30. Masaki T, Qu J, Cholewa-Waclaw J, Burr K, Raaum R RA. Leprosy Bacilli Promotes Dissemination of Infection. Cell. 2015;152(0):51–67.
31. Azevedo M de CS, Marques H, Binelli LS, Malange MSV, Devides AC, Silva EA, et al. Simultaneous analysis of multiple T helper subsets in leprosy reveals distinct patterns of Th1, Th2, Th17 and Tregs markers expression in clinical forms and reactional events. Med Microbiol Immunol. 2017;206(6):429–39.
32. Wagenaar I, Post E, Brandsma W, Bowers B, Alam K, Shetty V, et al. Effectiveness of 32 versus 20 weeks of prednisolone in leprosy patients with recent nerve function impairment: A randomized controlled trial. PLoS Negl Trop Dis. 2017;11(10):1–18.
- Log in to post comments